Radium Decay & Nuclear Reactions: A Comprehensive Guide - Explained!
Is there a hidden world of instability lurking within the very atoms that make up our reality? The answer lies in the realm of radioactive decay, where elements like radium shed particles and energy, transforming themselves in the process.
Radium, a fascinating element, holds a unique position in the periodic table. Designated as element number 88 (Ra), it's a member of the alkaline earth metals, but unlike its stable counterparts, radium exists in a perpetual state of flux. Its unstable nature stems from the inherent properties of its atomic nucleus, a tiny powerhouse of protons and neutrons. These nuclei are constantly striving for a more stable configuration, leading to a variety of decay processes. Radium's instability leads to the emission of alpha and beta particles, accompanied by gamma radiation, and the creation of radon gas. This intricate dance of particles and energy underscores the dynamic nature of matter at its most fundamental level.
Let's delve deeper into the properties of radium. One of the critical aspects to understand is that radium lacks stable or nearly stable isotopes. This inherent instability means that we cannot provide a standard atomic weight for this element in the traditional sense. The atomic weight of an element is usually calculated based on the relative abundance of its stable isotopes. Since radium lacks these, its atomic mass is a more complex value, reflecting the average mass of the radioactive isotopes.
The journey of radium through radioactive decay can be visualized through a balanced nuclear equation. In the case of alpha decay, a common occurrence, a radium-226 isotope (226/88Ra) transforms into a radon-222 isotope (222/86Rn), along with the emission of an alpha particle, which is essentially a helium nucleus (4/2He). This equation, representing the transformation, adheres to the laws of conservation, ensuring the mass number (total number of protons and neutrons) and the atomic number (number of protons) are balanced on both sides of the equation. The equation looks like this: 226/88Ra 4/2He + 222/86Rn
Radium-226, a common isotope of radium, has a half-life of approximately 1620 years. This half-life denotes the time it takes for half of the atoms in a sample to undergo radioactive decay. It's a stark reminder of the relentless process within these atoms, gradually transforming. With a half-life measured in millennia, radium's decay is a slow, but persistent process.
The emission of gamma rays adds another layer of complexity to radium's decay. Gamma rays are high-energy photons, electromagnetic radiation emitted from the nucleus of an atom during radioactive decay. They often accompany the emission of alpha or beta particles, carrying away the excess energy. The simultaneous emission of a beta particle and a gamma ray is a typical occurrence in certain radioactive decay processes. Gamma radiation is also not typically written in nuclear equations because they do not affect charges, atomic numbers, or mass numbers.
The transformation of radium through alpha decay provides valuable insights into nuclear physics. When a nucleus of radium-226 emits an alpha particle, the resulting nucleus has a mass number of 222, derived by subtracting the mass of the alpha particle (4) from the initial mass number (226). The atomic number decreases by two as the nucleus sheds two protons in the alpha particle. In the specific case of radium-226, the daughter element is radon (Rn), with an atomic number of 86.
Let us consider the energy released during alpha decay using the Q value. The Q value is the energy released during the decay process. When a 226/88Ra nucleus emits a 4.60 MeV alpha particle 5.5% of the time when it decays to 222/86Ra the Q value for this decay. Q = 4.68 MeV.
The concept of transmutation is essential when studying radioactive decay. Transmutation is the transformation of one element into another due to a change in the number of protons within the nucleus. Alpha and beta decay are primary examples of transmutation processes, and understanding these is critical for understanding how an atom transforms its identity. The element radium is a perfect example of how one element can transform into a different element.
Nuclear reactions have distinct characteristics compared to ordinary chemical reactions. In nuclear reactions, the atomic nuclei themselves undergo changes, leading to the formation of entirely new elements. In contrast, chemical reactions primarily involve the rearrangement of electrons, and the atomic nuclei remain unchanged. The balanced equations for nuclear changes must account for the conservation of mass number and atomic number, which is not necessarily a factor in chemical equations.
For those seeking to deepen their knowledge, platforms like Quizlet provide opportunities to study and memorize flashcards on key terms like the element radium. These resources are valuable for mastering the fundamentals of this dynamic field.
The journey through the world of radium reveals a lot about the laws of nature. It also makes us understand the world more.
Characteristic | Details |
---|---|
Name | Radium |
Symbol | Ra |
Atomic Number | 88 |
Mass Number (most common isotope) | 226 |
Radioactive Decay Modes | Alpha and Beta Emission |
Half-life (Radium-226) | 1620 years |
Emissions | Alpha particles, beta particles, gamma rays, Radon gas |
Discovery | Marie and Pierre Curie (1898) |
Location in Periodic Table | Group 2, Period 7 |
Uses | Historical: Used in medical applications and luminous paints. Today: Used in industrial radiography and some cancer treatments. |
Reference Website | Britannica - Radium |
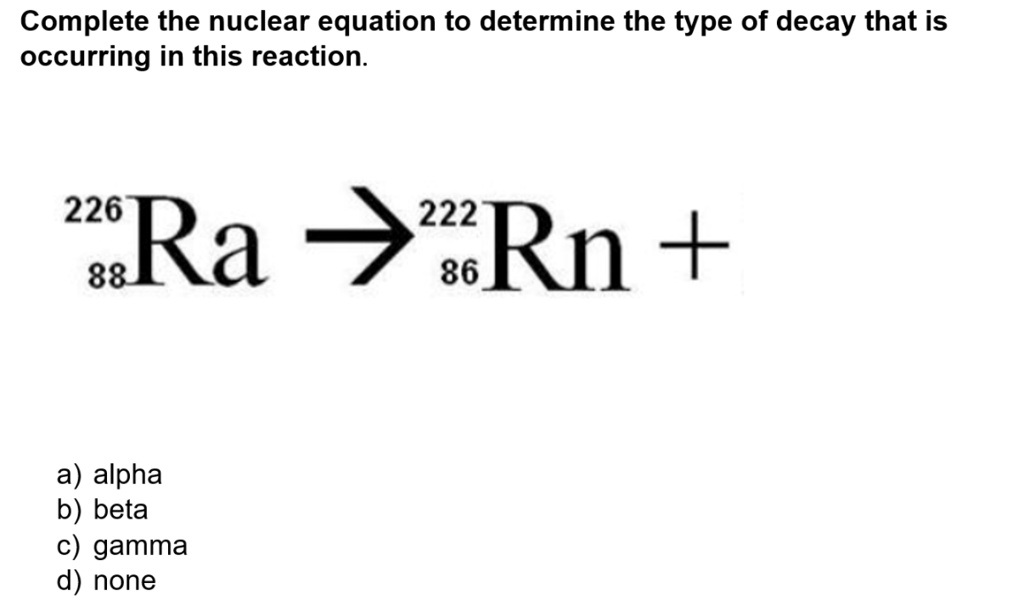
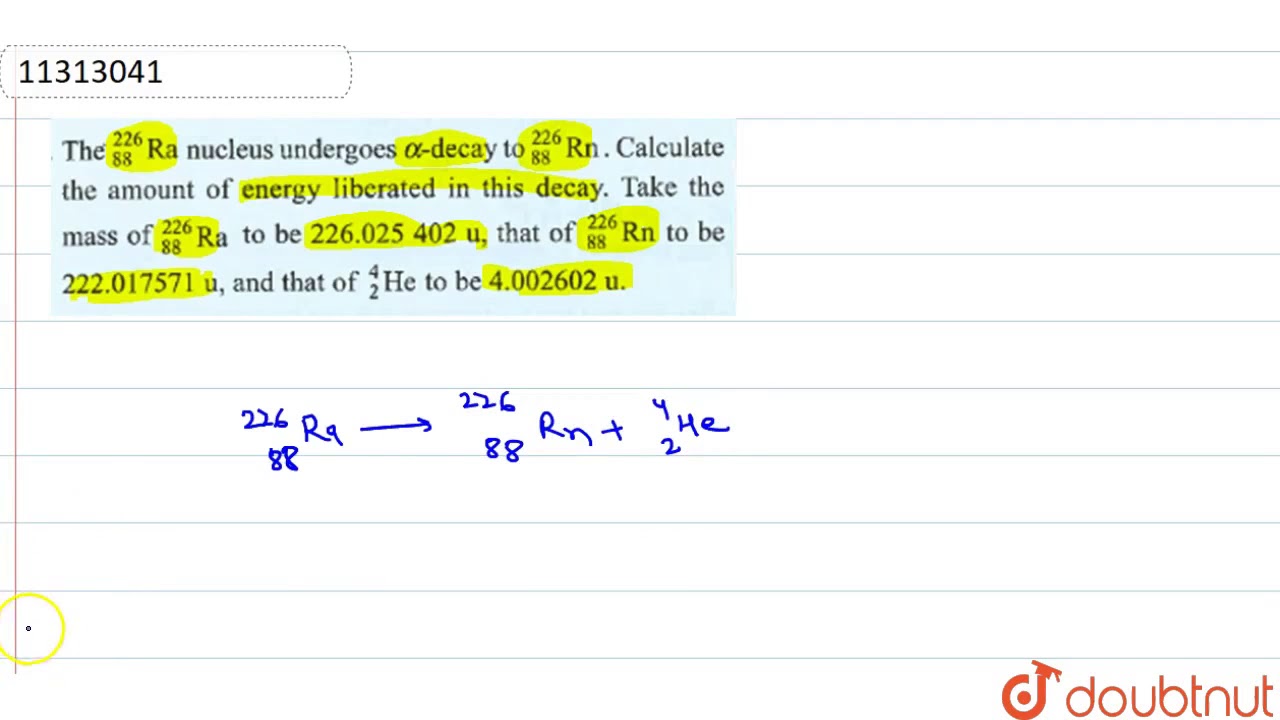
